Introduction
Room temperature air is made up of primarily nitrogen and oxygen molecules. However, as the temperature is increased to high temperatures, such as the temperatures experienced during hypersonic flight and re-entry flows, the gas can become chemically reactive. For example, hypersonic flight is characterized by very strong, oblique shocks as illustrated below.

As the speed is increased, the oblique shock wave moves closer to the surface and the shock layer becomes very hot. Aspects of such high-temperature flows are that they are chemically reactive (i.e. dissociation and ionization of the gas) and often in thermal nonequilibrium. These effects largely originate from the amount of energy in the flow. Flows at these high velocities inherently have a large amount of kinetic energy, which is converted to heat as it slows down and travels around the vehicle. Although the strong shock is oblique, the shock will be approximately normal near the leading edge.
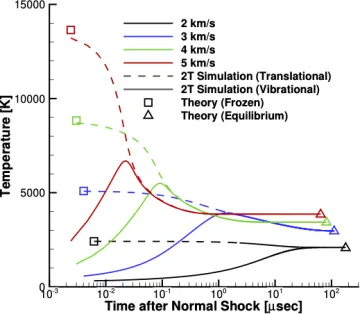
The figure above uses normal shock relations to approximate the temperature increase across the shock near the leading edge for different altitudes. The calorically perfect gas uses ideal gas relations and the chemically reacting gas account for real gas effects using NASA's Chemical Equilibrium with Applications (CEA) computer program. The chemically reactive flow is represented by two limits: equilibrium and frozen. An equilibrium chemically reactive flow allows for energy transfer between energy modes and the flow to reach a steady-state (i.e. equilibrium). There is no energy transfer between energy modes for frozen chemically reactive flows (i.e. very nonequilibrium). The actual temperature increase behind the normal shock will lie between these two limits as the energy transfer between modes depends on the time-scale. The importance of modeling nonequilibrium is illustrated by the large difference between the two limits. By any account, the temperature increase across the shock in the nose region of a hypersonic vehicle is extremely high, leading to high-temperature flows very near the leading edge.